Cytometry, encompassing flow cytometry and mass cytometry, has revolutionized our understanding of cellular complexity. These powerful technologies enable researchers to deeply profile individual cells, revealing a wealth of information about their phenotype, function, and interactions. This guide provides a comprehensive overview of cytometry, focusing on its capabilities, limitations, and applications, particularly for those seeking a “deep profilers guide to cytometry”.
The Power of Single-Cell Analysis
Unraveling the intricacies of biological systems requires a deep understanding of cell behavior and their mechanisms. Perturbations, whether innate or infectious, can drive disease pathogenesis. “Deep profiling” analyzes cells at the single-cell level, encompassing RNA, protein, and glycan species, across a large number of cells, this approach offers a comprehensive understanding.
Flow cytometry stands out for its rapid interrogation of multiple biologic signatures (protein epitopes, nucleic acids, ion concentrations) within individual cells. Over the past four decades, the maximum number of measurable proteins has steadily increased, driven by advancements in hardware, fluorochromes, and data analysis. Polychromatic flow cytometry (PFC, 6+ colors) has become a workhorse in immunology and cell biology. Furthermore, mass cytometry has emerged as a “post-fluorescence” single-cell technology capable of measuring 70–100 parameters. These technologies offer unique features and address different questions, playing central roles in dissecting the intricate interactions of cells.
The Polychromatic Revolution
Polychromatic flow cytometry’s evolution depended on stepwise progress in hardware and reagents. Early cytometers used broad-spectrum arc lamps that interfered with fluorochrome signals. The Herzenberg lab utilized argon lasers emitting a single wavelength (488 nm) for fluorescein, increasing sensitivity. Krypton lasers were added for two-color detection. Water-cooled lasers were replaced with HeNe lasers and solid-state lasers, ideal for exciting phycobiliproteins like phycoerythrin (PE) and allophycocyanin (APC).
PE’s ability to transfer energy to other fluorescent molecules led to tandem dyes (e.g., PE-Texas Red, PE-Cy5). APC-based tandem dyes and Alexa dyes became available in the 1990s. Violet (405 nm) lasers emerged alongside fluorescent, inorganic semiconductor nanocrystals (Quantum Dots) in 2004, leading to the 18-color cytometry. Organic polymers that conduct electrons provided additional violet-excitable fluorochromes.
Technical Achievements of PFC
18-color flow cytometry resulted from achievements in laser and fluorochrome technology, and engineering advances in optics and signal processing. Software development for rapid data processing and fluorescence spillover correction (“compensation”) was also important. Tools for displaying and analyzing 20-parameter data across samples have been developed. PFC advancements also required chemistry (dye development), hardware, and software improvements.
Applications of PFC
Technical advances in flow cytometry have deepened our understanding of hematopoietic cell types and function. Early cytometers resolved major cell lineages like T- and B-cells, allowing characterization of immunity. Three-color measurements of T-cell subsets (CD4+ helper and CD8+ cytotoxic) became important due to monoclonal antibodies and the HIV epidemic. Leukemia and lymphoma typing now relies on flow cytometry, now using 8–10 for better fidelity. In the 1990s, naive and memory T-cell subsets were distinguished, and cytokine production was quantified. PFC instrumentation gives unprecedented resolution of immune system cells.
The utility of 15-color experiments is shown by the identification of memory T-cells with stem-like properties (“TSCM”). TSCM have proliferative capacity and mediate anti-tumor responses. As designing complex staining panels is difficult, even more measurements are required to characterize multiple chemokine receptors on TSCM. Barcoding schemes distinguish cells from different samples in a mixture, requiring additional parameters. Meeting the need for higher levels of multi-parametric analysis of single cells requires new technologies to complement PFC.
Entering the Post-Fluorescence Era: Mass Cytometry
Mass cytometry, couples flow cytometry with mass spectrometry. This technology offers single-cell analysis of at least 45 simultaneous parameters without fluorescent agents or spectral overlap. Stable isotopes of rare earth metals are reporters. Mass spectrometry’s resolution, sensitivity, and dynamic range on a time-scale that allows the measurement of 1000 cells per second, offers high content cytometric analysis.
Elemental Mass Spectrometry
Inductively coupled plasma mass spectrometry (ICP-MS) determines the elemental composition of materials. It has been used for trace detection of metals in environmental and clinical samples. The system utilizes a high-temperature plasma (~7000 K) that vaporizes the sample, breaks bonds, and strips atoms of electrons. Elemental ions are introduced into an ICP-MS for relative abundance determination. ICP-MS is suited for bioanalysis because it can simultaneously detect trace-levels of non-biological elements from complex matrices.
Mass Cytometry Techniques
Mass cytometry adapts ICP-MS to single-cell analysis, based on using a purified single isotope to tag antibodies quantified in an ICP-MS system. Mass cytometry follows the same workflow as flow cytometry. Cells are stained with metal isotope-labeled antibodies (typically lanthanide metals). Cells are also stained with DNA intercalators and viability measures.
In the instrument, stained cells are nebulized into single-cell droplets and introduced into the plasma. The charged atomic ion clouds are immediately transferred into the high vacuum of the mass spectrometer. A quadrupole-time-of-flight (qTOF) instrument is configured to resolve lanthanides from ions.
The ion cloud has a lifetime of ~300 μs, during which it is measured 20–30 times by TOF-MS. Measuring >1000 cells per second would result in inability to resolve single cells. Since elemental reporters are rare in a biological context, there is little background signal between cells. The amount of each isotopic reporter is quantified for each cell’s ion cloud and recorded in an FCS file format. The CyTOF mass cytometer and associated reagents are produced and distributed by DVS Sciences.
Mass Cytometry vs. PFC: A Comparative Analysis
Mass cytometry offers unique features compared to PFC, but it also encompasses unique hurdles. PFC can work on live cells, and analyzed cells can be recovered. While the two technologies overlap, each addresses a particular set of questions.
Technology | Fluorescence Flow Cytometry | Mass Cytometry |
---|---|---|
Measurement basis | Fluorescent probes | Stable mass isotope probes |
Experimental design | ||
Max # of measurements | 20 (18 fluorescence) | 37 (including DNA) |
Theoretical # of subsets | 2.6 × 105 | 1.4 × 1011 |
Panel design complexity (# of probes) | Easy: <8 | Difficult: 37 |
Moderate: 8–12 | ||
Hard: 12–18 | ||
Sensitivity range for different probes | 0.1 – 10 | 1 – 2 |
Sample Throughput | ||
Sampling Efficiency | > 95% | < 30% |
Measured Cells/second | 25,000 | 500 – 1,000 |
Cells/hour | 25 – 60 million | 2 million |
Commercial reagent cost | ||
Per probe per test | $2 – 8 | $1.50 – 3 |
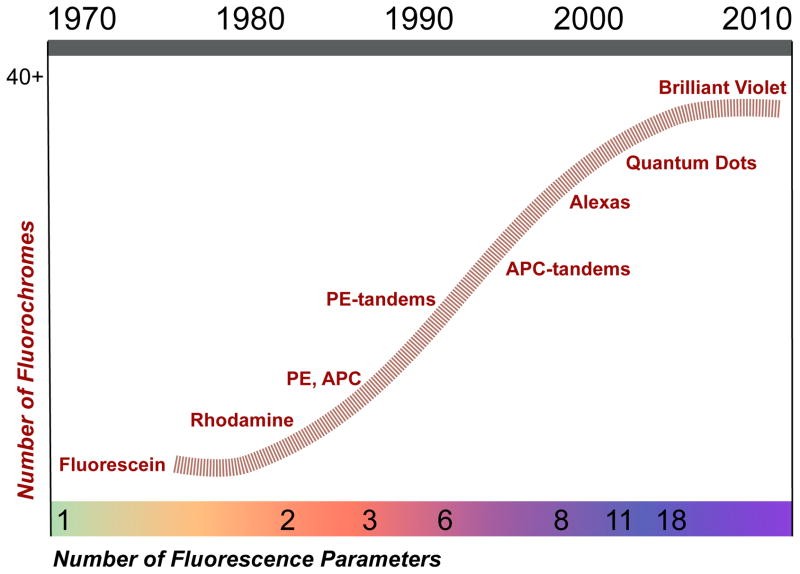
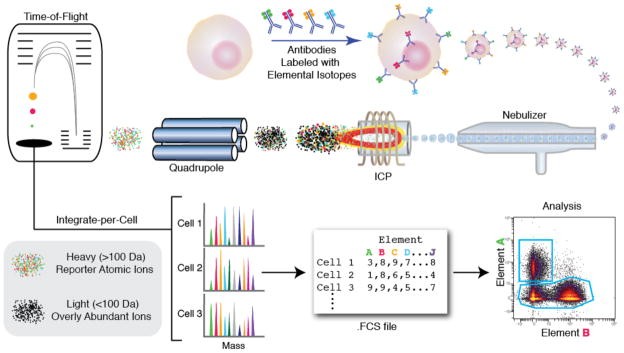
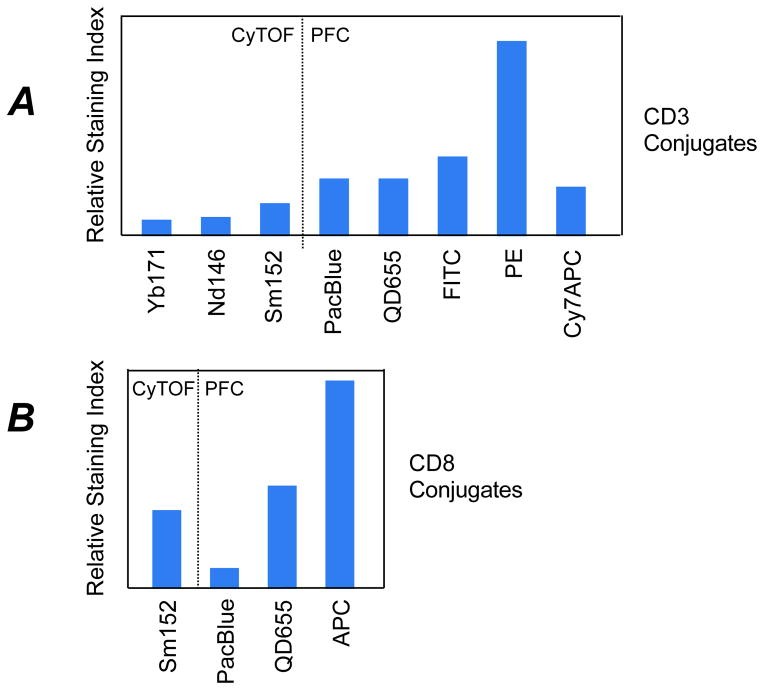
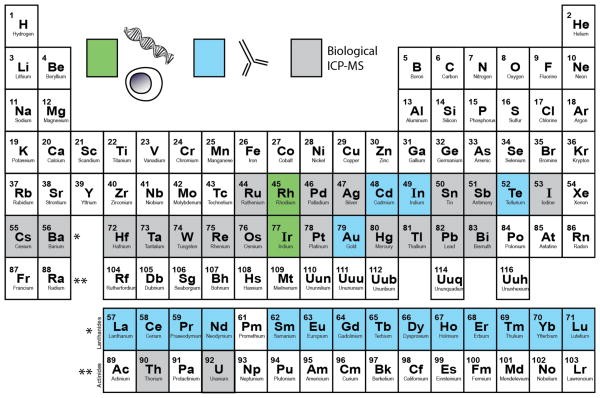
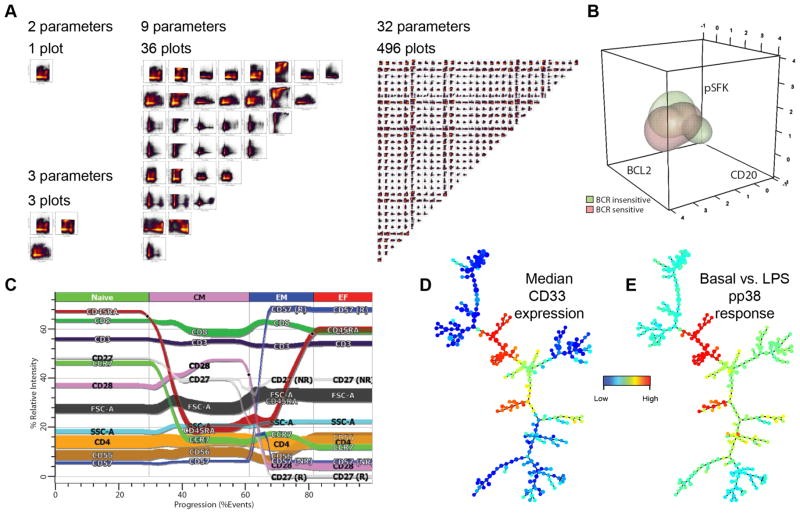
Dimensionality in Cytometry
For large-scale mass cytometry experiments, learning curves are not steep due to PFC principles. Regulatory cell signaling behavior across hematopoietic cells was examined using two 34-parameter panels. The study included 31 antibody targets, a DNA intercalator, and measures of viability and cell size. One panel employed 31 channels for cellular phenotyping, while the other simultaneously analyzed intracellular phosphorylation responses. A 37-parameter study of virus-specific T-cell function and phenotype was reported in less than a year. Mapping the immune system requires a set of 12+ markers and fluorophores. PFC panels become laborious as the number of markers reaches 15, due to the 18 marker limit. In cases in which multiple intracellular events must be tracked, PFC cannot simultaneously detail multiple pathways across cell subsets.
Mass cytometry can measure many parameters without sensitivity loss. Mass cytometry cannot measure forward and side scatter (FSC and SSC), cell size, and granularity which is used to discriminate large granular leukocytes, lymphocytes, doublets of cells, and cellular debris. Also, small molecule fluorescent reporters for Ca2+ flux, mitochondrial permeation, and cell division (CFSE) do not have metal-reporter equivalents. There are opportunities to measure metal parameters at the single-cell level.
Sensitivity Comparisons
The sensitivity of lanthanide-tagged antibodies is lower than fluorescent reporters. The chelating polymer common to probes limits metal reporter ions attached to the antibody molecule. Low background signals in mass cytometry can balance this deficiency in the measurement of subtle regulatory changes (e.g. protein phosphorylation). The sensitivity of lanthanide-based reporters varies by 2-fold. The sensitivity of fluorochromes can differ widely (10–50 fold) in flow cytometry.
Usability of Mass Cytometry
The CyTOF mass cytometer has three orders of magnitude of resolution between detection mass channels. The signal response for lanthanide metal isotope reporters falls within 2-fold of one another. Mass cytometry simplifies experimental panel design because changing a single parameter might not require redesign of the entire analysis panel to avoid spectral overlap issues. Mass cytometry all but eliminates spectral overlap issues, but isotopic impurities and oxidation of reporter ions can still cause signal interference/overlap.
Quantitation of Cells
Both fluorescence and mass-based measurements are quantitative with proper controls. The CyTOF mass cytometer is linear across almost four orders of magnitude. Fluorescence flow cytometers typically have a five order of magnitude range where linearity depends on the photon amplifiers. Autofluorescence and spectral spillover compromise the working dynamic range for fluorescence detection. Fluorescence measurements will be somewhat better than mass cytometry in theory; this precision is better than biological variation.
Sampling and Throughput in Cytometry
Mass cytometry throughput is limited to about 1000 cells per second. Commercial fluorescence cytometers can operate at rates 25–50 times faster. Cell injection and cleaning routines are time-consuming, and the nebulization of single cell droplets into the ICP is inefficient. The current generation mass cytometer can process 8 samples per hour, with 250,000 processed events per sample.
Experimental Mass Cytometry
Mass cytometry is best for investigating intracellular regulatory molecules or for a very high number of simultaneous measurements are needed. Fluorescence cytometry may be better suited for rare event analysis, phenotyping requiring measurement of low abundant cellular targets or light-scatter properties, and the rapid analysis of individual samples. Improvements are DNA branch chain techniques, polymers with more chelator sites, lanthanide nanocrystals, and more efficient sampling interfaces with integrated optics.
Future Directions in Cytometry
Improvements in mass cytometry should dramatically increase its utility and are focused on throughput, mass and dynamic range, and development of additional and more sensitive metal isotope probes. Introducing a prototype auto-sampler will decrease wash-out time between samples and allow automated acquisition. In flow cytometry, fluorescent cell barcoding (FCB) is used as a sample multiplexing technique. Barcoding can be adapted to mass cytometry to allow up to 128 conditions to be multiplexed using seven parameters.
Commercial ICP-MS instruments have a range of 109, but the CyTOF mass cytometer range is 104. The limitation is in the speed of computer hardware. Without digitization limitations, the current CyTOF mass cytometer could measure non-biological transition metals. Future generations of instrumentation should be much improved.
There are enough non-biological elemental isotopes to move mass cytometry measurements beyond 50 simultaneous parameters per cell. Attaching reporter isotopes to antibodies and the availability of pure forms of additional isotopes are required for the effort. The utilization of chelators targeting metal ions and isotopes can provide additional measurement parameters.
Sensitivity of mass cytometry instrumentation, and reagent technology are expected to improve. Commercial chelating polymers allow the attachment of 100 metal atoms per antibody. Probes can be constructed with substantially higher metal content. Solid metal nanoparticles can serve as reporters in mass cytometry and contain 105 atoms of a metal. Optimized application could increase the number of atoms bound per antibody, lowering the detection limit, increasing signal intensity, and better resolving cell populations with low target protein expression.
Analysis of Multiplexed Data
Increases in the measurable single-cell parameters in flow and mass cytometry are daunting. Analysis of flow cytometry data is manual, which suffers from user bias. Unexpected cell types may be overlooked because analysis requires knowledge of the cell-type of interest.
Flowjo simplifies and standardizes multi-sample analyses and contains graphical outputs for visually summarizing multi-dimensional experiments. Cytobank is a collaboration-centric, web-based analysis platform. SPICE helps identify trends across user-defined cell populations in study groups.
Approaches are required to enable ‘human interpretable’ two-dimensional visualizations that require managed user supervision.
Samspectral and density reduction clustering cluster cells based on the expression levels of parameters, but SPICE does not directly compare these clusters across sample sets. FLAME performs unsupervised clustering, providing tools to visually compare clusters between samples. Spanning-tree progression analysis of density-normalized events (SPADE) clusters cells in multidimensional space and then reduces them to a two-dimensional representation using a minimum spanning tree algorithm.
Principle components analysis (PCA) is not generally useful to immunophenotyping data. FlowType takes data, automatically defines populations, exhaustively stratifies all possible combinations of markers, and compares them across different samples. SPADE and similar packages are available through Bioconductor and being evaluated using datasets against performance metrics. Also, the parameters expressed by a single cell can be linked to each other to construct relationship networks. This approach, which employs Bayesian inference, has been particularly useful to examine T cell receptor signaling and investigating cell signaling feedback mechanisms.
Gemstone works to create a 2D summary of single cell datasets – exploiting the expression patterns of parameters. It employs probability state modeling to organize cell populations.
Many of these approaches consider that the measurement of multiple markers can provide information for understanding a disease. Given that cells can be parsed too finely, it is important to consider the number of parameters that must be measured. High dimensional, hypothesis generating experiments, and the tools to analyze and distill them, will be necessary.
Conclusion: The Future of Cytometry
Continual improvement in single-cell analysis technologies has driven the investigation and understanding of immunology and stem cell biology. Pushing the limits of fluorescence-based analysis has led to unprecedented studies of regulatory signaling. Next-generation, mass cytometry instrumentation will likely drive “deep profiling” in mapping biological mechanisms, the role of multiple cell subsets, and how malfunctions cause disease. Fluorescence and mass cytometry will continue to be critical tools, their complementarity with other single-cell applications has promise for discovery.